Glacier mass balance | Glacier flow | Internal deformation | Basal sliding | Subglacial deformation | Different types of glacier flow | References | Comments |
Glacier mass balance
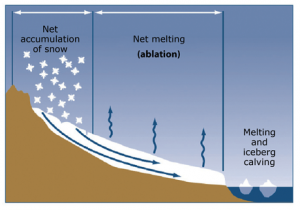
How do glaciers move? A glacier is a pile of ice, and as such, deforms under the force of gravity. Glaciers flow downslope because they accumulate mass (ice) in their upper portions (from precipitation and from wind-blown snow) and ablate (melt, sublimate and calve ice bergs) in their lower portions.
This means that a glacier in a steady state (equilibrium) will not change in steepness or size, because accumulation = ablation. The altitude with zero net accumulation or ablation on the glacier is the equilibrium line altitude. Changes in rates of accumulation or ablation will lead to glacier advance or recession; if the accumulation area of a glacier shrinks, for example, and the equilibrium line altitude rises, then the glacier will recede.
Glacier mass balance is the difference between accumulation and ablation. It is therefore controlled by both temperature and precipitation. If accumulation is greater than ablation, then the glacier has positive mass balance and will advance. If ablation is greater than accumulation, then the glacier has negative mass balance and will recede.
Note: see Common Misconceptions in Glaciology. Glaciers always flow downslope under the weight of their own gravity. A receding or shrinking glacier still flows (although it might flow very slowly!); it’s just that it’s melting faster than it’s acquiring snow in its upper reaches. As a result, the glacier will thin and the snout position will recede backwards.

In theory, glaciers discharge ice from the accumulation area to the ablation area and maintain a steady-state profile. The velocity, the balance velocity, is controlled by glacier mass balance and glacier geometry (Jiskoot et al., 2011). Some glaciers have dynamic flow driven by other factors, for example, surging glaciers, tidewater glaciers, ice streams or ice-shelf tributary glaciers.
Glaciers flow through ice deformation and sliding
Glaciers always flow downslope, through the processes of deformation and sliding. Glacier flow, velocity and motion is controlled several factors (Jiskoot et al., 2011), including those listed below:
- Ice geometry (thickness, steepness),
- Ice properties (temperature, density),
- Valley geometry,
- Bedrock conditions (hard, soft, frozen or thawed bed),
- Subglacial hydrology,
- Terminal environment (land, sea, ice shelf, sea ice), and
- Mass balance (rate of accumulation and ablation).
When glaciers flow downslope, gravitational driving stresses are resisted (resistive stress). The driving stress is controlled by gravitational acceleration, ice density and temperature, ice thickness and ice surface slope. Resistive stresses mainly operate at the glacier bed, and comprise basal drag or lateral drag against valley walls.
This driving stress means that glaciers move in one of three ways:
- Internal deformation (creep)
- Basal sliding
- Soft bed subglacial deformation.
All glaciers flow by creep, but only glaciers with water at their base (temperate or polythermal – see Glacial Processes) have basal sliding, and only glaciers that lie on soft deformable beds have soft sediment deformation. If all three factors are present, you have the ingredients to contribute to fast ice flow (see Ice Streams).
Internal deformation
If the glacier flows just by internal deformation, then it is likely that rates of creep decrease with depth, with fastest ice movement at the surface and slowest (or no) ice movement at the base and at the valley sides, where resistive stresses are highest (Jiskoot et al., 2011). Ice deforms because it is plastic. If large stresses are applied it can crack in a brittle manner (forming crevasses or calving ice bergs).
The video below shows a huge calving event at Helheim Glacier, Greenland, in July 2010. It was made by the Swansea Glaciology Group.
Basal sliding
Glaciers can slide because ice melts under pressure, resulting in a film of water at the ice-bed interface. This can facilitate decoupling and enhance fast ice flow. If the glacier bed is rough, with many bumps and obstacles, this increases melting and ice flow. This process is known as regelation. If water pressures become high enough, cavities can form at the ice-bed interface, causing sliding with bed separation. This reduces basal friction and allows faster ice flow. Sliding velocity is controlled by basal shear stress and effective pressure, which is the difference between ice overburden pressure and water pressure (Jiskoot et al., 2011).
This video shows the glacier flowing above a cavity beneath the ice on Mont Blanc glacier.
Subglacial deformation
Subglacial till (see Glacial Processes) comprises unconsolidated, unsorted or poorly sorted sediments ranging from boulders to clay. In Norfolk, till sequences are over 20 metres thick. Fine sediments, such as clay and sand, are not cohesive and therefore deform readily when shear stress is applied to them if they have a high pore-water pressure (so, like basal sliding, subglacial deformation depends on high basal water pressures). If basal shear stress (the gravitational driving dress) is greater than the yield strength of the till, deformation occurs, resulting in some fantastic glaciotectonic sequences (see picture gallery below and papers by Davies et al., 2009, 2012a, 2012b).
Different types of glacier flow
Glaciers do not just flow in a steady state, however. We have cold-based glaciers, which have little flow velocity; polythermal glaciers, which are partly frozen to their bed; wet-based glaciers which have sheet flow (as is described in the above sections); ice streams, which have very rapid flow velocities; and surging glaciers, which have periods of rapid flow separated by quiescent periods of slow flow. The key differences in temperate glacier flow is summarised in the table below. Each of these different flow regimes results in a set of different and diagnostic glacial landforms.
Further Reading
- Glacier Flow II: Stress and Strain
- Glacial thermal regime
- Types of Glacier
- Ice streams
- Glacial hydrology
Other sources:
Go to top or jump to Glacier thermal regime.
Go to top or jump to Glacier thermal regime.
Pingback: How Does a Glacier Move? | Matt Chernos
Pingback: What’s happening under that glacier?! | The road to a PhD...
this page really help me a lot in glacier basics, thank the authors!
thanks!
How do I cite this article
how is it possible to write about these topics without citing Nye or Glen???
this is an introductory level post, and the citations are appropriate.
Scientists take ice cores from glaciers. How do we know if the ice cores are compromised from flowing over structures, snow falling into crevasses, crevasses collapsing into themselves or becoming plastic under pressure, or high winds clear certain potential layers and accumulate elsewhere to form additional layers? How do we know if intermittent blizzards and warm days occurring within a single year might appear as multiple-year rings in ice cores?
Hello,
Taking an ice core is a long, arduous and expensive process. Therefore the first stage is always an extensive survey. Techniques such as radar allow the layers in ice to be mapped and visualised regionally, allowing deformation of ice to be observed and accounted for. Generally ice cores are taken from as close to the ice divide as possible, where there is very little flow and the flow is away from the point in all directions.
Geochemical signatures give predictable and measurable seasonal patterns that can be linked to visual layers in the ice. However the layers are usually measured based on seasonal isotopic variations.
Melt layers do occur within summer layers in some parts of the world, depending on where the ice core was taken. These are observable within the visual layers of the ice.
Hello,
Thanks for the nice post. i am a little confused. maybe i think this statement is not exactly right in the Deformation section. “then it is likely that rates of creep decrease with depth”.
according to your reference Jiskoot (2011), the glacier veolocity due to deformation decreased with depth, not the creep rate. becasue the surface velocity accumulates all the deformation from every layer beneath it. near the glacier surface, creep rate may be lower because the ice is colder and less subject to pressure-induced derformation. near the base, basal ice is under greast stress and often near the melting point, making it more prone to deformation. An example is from Behn et al. (2021).TC. https://doi.org/10.5194/tc-15-4589-2021, 2021. Figure 6.